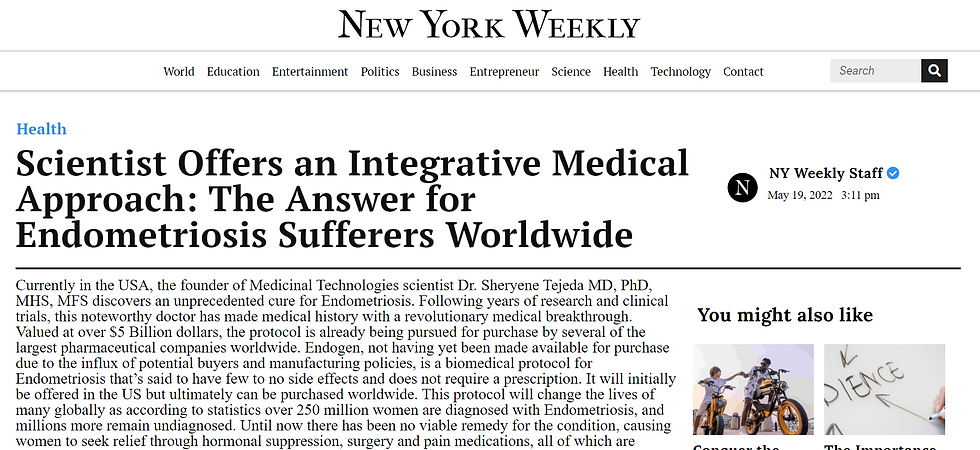
Sheryene Tejeda Biomaterials are integral to modern medicine, enabling advances in diagnostics, treatment, and tissue regeneration. These materials, which interact with biological systems, are designed to repair, replace, or enhance biological functions. Innovations in biomaterials are driving significant improvements in clinical outcomes across a range of applications. This article explores the latest advancements in biomaterials and their diverse clinical applications.
1. Types of Biomaterials
Biomaterials can be classified based on their origin and composition:
Natural Biomaterials: Derived from biological sources, such as collagen, hyaluronic acid, and chitosan. These materials often mimic the natural extracellular matrix and are used in tissue engineering and regenerative medicine.
Synthetic Biomaterials: Engineered in the lab, including polymers like polylactic acid (PLA) and polyglycolic acid (PGA), as well as ceramics and metals. Synthetic biomaterials can be customized for specific applications and often offer enhanced control over material properties.
Composite Biomaterials: Combination of natural and synthetic materials to leverage the benefits of both. These composites are used to improve mechanical strength, biocompatibility, and functional performance.
2. Innovations in Biomaterials
Recent innovations in biomaterials are expanding their applications and improving their functionality:
Smart Biomaterials: These materials respond to environmental stimuli, such as changes in pH, temperature, or light. Examples include:
Self-Healing Materials: Capable of repairing themselves after damage, extending the lifespan of implants and devices.
Responsive Hydrogels: Hydrogels that change their properties in response to external stimuli, useful for controlled drug delivery and tissue engineering.
Biodegradable Biomaterials: Designed to break down safely within the body over time, reducing the need for surgical removal. Applications include:
Bioabsorbable Stents: Used in cardiovascular procedures to provide temporary support to blood vessels before being absorbed by the body.
Resorbable Sutures: Used in surgical procedures to provide temporary support while tissue heals.
3D-Printed Biomaterials: Advances in 3D printing technology are enabling the creation of customized implants and prosthetics. Benefits include:
Patient-Specific Implants: Tailored to match the anatomical structure of individual patients, improving fit and function.
Complex Tissue Structures: 3D printing can produce complex tissue scaffolds for regenerative medicine, including bone, cartilage, and vascular tissues.
Nanomaterials: Engineered at the nanoscale to enhance their properties and interactions with biological systems. Applications include:
Nanoparticle-Based Drug Delivery: Targeted delivery of drugs to specific cells or tissues, improving efficacy and reducing side effects.
Nanostructured Implants: Enhanced surface properties for improved integration with biological tissues and reduced infection risk.
3. Clinical Applications of Biomaterials
Biomaterials are utilized in a wide range of clinical applications, including:
Tissue Engineering and Regeneration: Biomaterials serve as scaffolds for tissue regeneration and repair:
Bone and Cartilage Regeneration: Biomaterials such as synthetic ceramics and hydrogels support the growth of new bone and cartilage tissue, used in orthopedic and reconstructive surgeries.
Skin Substitutes: Natural and synthetic skin substitutes are used for treating severe burns and chronic wounds, promoting skin regeneration and healing.
Implants and Prosthetics: Biomaterials are essential for developing implants and prosthetic devices:
Orthopedic Implants: Materials such as titanium and cobalt-chrome alloys are used in joint replacements, bone fixation devices, and spinal implants.
Dental Implants: Biocompatible materials, including titanium and zirconia, are used for dental implants, providing a stable foundation for artificial teeth.
Drug Delivery Systems: Biomaterials facilitate controlled and targeted drug delivery:
Microspheres and Nanoparticles: These systems release drugs in a controlled manner, improving therapeutic outcomes and minimizing side effects.
Implantable Drug Reservoirs: Biomaterials used in implantable devices provide sustained drug release over extended periods.
Wound Healing: Advanced biomaterials support wound healing and tissue repair:
Bioactive Dressings: Incorporate growth factors, antimicrobial agents, and other therapeutic components to enhance wound healing.
Hydrogel Dressings: Provide a moist environment that promotes healing and protects wounds from infection.
Diagnostic Devices: Biomaterials are used in diagnostic applications to improve accuracy and functionality:
Biosensors: Biomaterials used in biosensors detect specific biomarkers or pathogens, enabling early diagnosis and monitoring of diseases.
Imaging Agents: Advanced biomaterials serve as contrast agents in imaging techniques, enhancing the visualization of tissues and abnormalities.
4. Challenges and Future Directions
While biomaterials offer significant benefits, several challenges and future directions need to be addressed:
Biocompatibility and Safety: Ensuring that biomaterials do not elicit adverse reactions or toxicity is crucial. Ongoing research focuses on improving biocompatibility and minimizing inflammatory responses.
Long-Term Performance: The long-term performance and durability of biomaterials, especially in load-bearing applications, require continuous evaluation and improvement.
Regulatory and Ethical Issues: The development and use of biomaterials must navigate regulatory requirements and ethical considerations, particularly in areas such as genetic engineering and human tissue use.
Personalized Medicine: Advances in biomaterials will increasingly support personalized medicine approaches, with materials tailored to individual patients’ needs and conditions.